
Introduction to Polylactic Acid (PLA)
Bio-based
Bio-based plastics are distinguished from petroleum-based plastics by the initial source of the material.
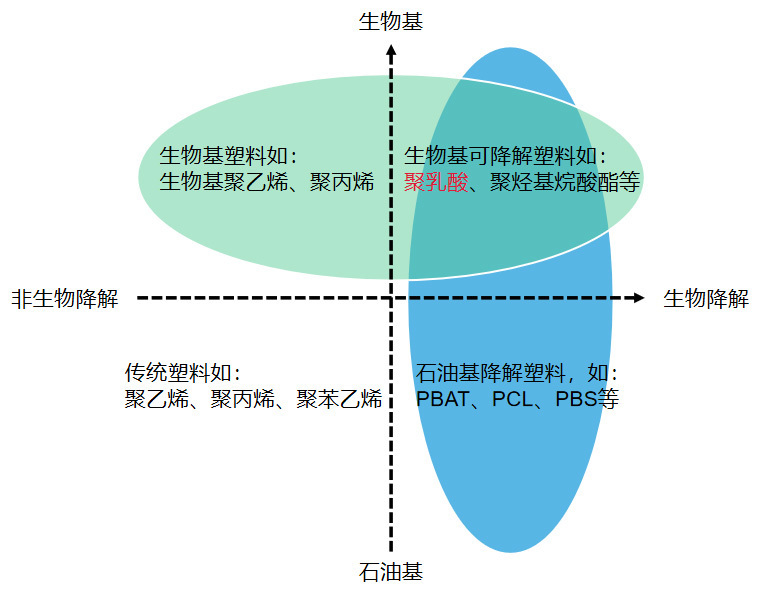
Polylactic acid
Future Development Directions of Biobased Materials
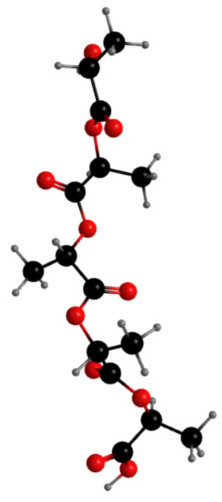
Future Development Directions of Biobased Materials

Bio-based

Polylactic acid

Key Features of PLA

Breakthrough in “Bottlenecks” Technologies
Product Application
Poly Lactic Acid, referred to as PLA, also known as polylactide
Product Advantages
Poly Lactic Acid, referred to as PLA, also known as polylactide
Brand List
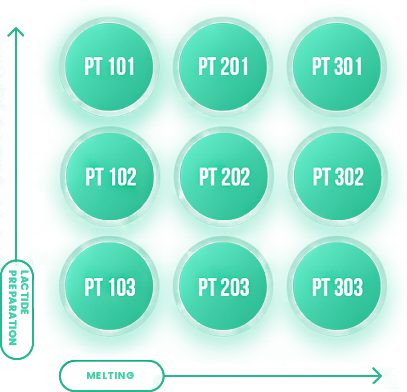
PLIITH PLA Materials can be processed by extrusion, stretching, spinning, injection molding, blow molding, blister forming, film blowing, and other molding processes for use in various fields.
New Catalytic System
① The invention solves the technical problem of low catalytic efficiency and racemization of stereoscopic structure during the oligomerization and cracking of lactic acid to prepare lactide and its polymerization, with high product yield.
② It solves the engineering problems of mass and heat transfer of high-viscosity, low-thermal-conductivity fluids under high-temperature conditions, achieving perfect integration of process and equipment, resulting in products with white color and excellent performance.
Project | Unit | PT 101 | PT 102 | PT 103 |
Density | g/cm3 | 1.24 | 1.24 | 1.24 |
Melt flow index | g/10min(190℃,2.16kg) | 3 | 3 | 3 |
Optical purity | % L-isomer | >99% | 98% | 96% |
Melting point | ℃ | 170-180 | 160-169 | 150-159 |
Glass transition temperature | ℃ | 55-60 | 55-60 | 55-60 |
Tensile modulus | MPa | 3500 | 3500 | 3500 |
Tensile strength | MPa | ≥50 | ≥50 | ≥45 |
Elongation at break | % | ≥3 | ≥3 | ≥3 |
Notched izod impact strength | kJ/m2 | ≥2 | ≥2 | ≥2 |
Heat deflection temperature (unreinforced) | ℃ | 50-60 | 50-60 | 50-60 |
Application direction | / | Heat-resistant straws, heat-resistant blister packaging, heat-resistant blow bottles, biaxially oriented films, 3D printing, spinning, modified materials | 3D printing consumables, film blowing, sheets, staple fibers, biaxially oriented films, modified materials | Film blowing, coating, cast film, staple fibers, spinning, 3D printing consumables, modified materials |
The data listed in the table are typical values only for reference during use and do not constitute product standards.
Project | Unit | PT 201 | PT 202 | PT 203 |
Density | g/cm3 | 1.24 | 1.24 | 1.24 |
Melt flow index | g/10min(190℃,2.16kg) | 10 | 10 | 10 |
Optical purity | % L-isomer | >99% | 98% | 96% |
Melting point | ℃ | 170-180 | 160-169 | 150-159 |
Glass transition temperature | ℃ | 55-60 | 55-60 | 55-60 |
Tensile modulus | MPa | 3500 | 3500 | 3500 |
Tensile strength | MPa | ≥50 | ≥50 | ≥45 |
Elongation at break | % | ≥3 | ≥3 | ≥3 |
Notched izod impact strength | kJ/m2 | ≥1 | ≥1 | ≥1 |
Heat deflection temperature (unreinforced) | ℃ | 50-60 | 50-60 | 50-60 |
Application direction | / | Long fibers, staple fibers, blister packaging, injection molding, modified materials | Staple fibers, blister packaging, injection molding, modified materials, spunbond nonwovens | Staple fibers, modified materials, spunbond nonwovens |
The data listed in the table are typical values only for reference during use and do not constitute product standards.
Project | Unit | PT 301 | PT 302 | PT 303 |
Density | g/cm3 | 1.24 | 1.24 | 1.24 |
Melt flow index | g/10min(190℃,2.16kg) | 30 | 30 | 30 |
Optical purity | % L-isomer | >99% | 98% | 96% |
Melting point | ℃ | 170-180 | 160-169 | 150-159 |
Glass transition temperature | ℃ | 55-60 | 55-60 | 55-60 |
Tensile modulus | MPa | 3500 | 3500 | 3500 |
Tensile strength | MPa | ≥50 | ≥50 | ≥45 |
Elongation at break | % | ≥3 | ≥3 | ≥3 |
Notched izod impact strength | kJ/m2 | ≥1 | ≥1 | ≥1 |
Heat deflection temperature (unreinforced) | ℃ | 50-60 | 50-60 | 50-60 |
Application direction | / | Injection molding and thin-wall injection molding (heat-resistant products), melt-blown nonwovens, modified materials | Injection molding and thin-wall injection molding (non-heat-resistant products), melt-blown nonwovens, modified materials | Melt-blown nonwovens, modified materials |
The data listed in the table are typical values only for reference during use and do not constitute product standards.
A model of industry-university-research-application collaboration
The Company has established a cooperative relationship with the team of Academician Chen Xuesi from the Changchun Institute of Applied Chemistry of Chinese Academy of Sciences, and annually invests RMB 10 million in industrialization and applied technology development. At the same time, PLIITH, the Changchun Institute of Applied Chemistry of Chinese Academy of Sciences, and the Wuhu Municipal People's Government jointly build the "Wuhu Biobased Materials Joint Innovation Center", which will comprehensively focus on the technological research and development and industrialization of common technical difficulties, frontier technologies, green manufacturing, and product applications in the biobased materials industry.
Establish a core technology system for the entire industry chain
With lactic acid fermentation, high-purity lactide preparation, and lactide polymerization to produce polylactic acid (PLA) with high chemical purity, high optical purity, and narrow molecular weight distribution as the core technologies, the Company has fully mastered the entire "sugar-lactic acid-lactide-PLA" industry chain technology, achieving domestic and international technological leadership in the field of PLA.
Highly intelligent production line
Achieving fully automated production throughout the process, the project's production line has the capability to produce multiple grades on a single line, allowing for switching between resin grades based on production schedules. The production line can also achieve online addition of corresponding catalysts and additives, reducing human interference, resulting in higher efficiency, better product quality, and greater stability.
Profound technical reserves
Deployments have been made in areas such as the development of efficient compound catalytic systems, new methods for lactide synthesis, rapid crystallization PLA resins, and special resins for stereocrystalline PLA, forming a multi-level technical reserve that will accumulate and deliver significant results.
Breakthrough in “Bottlenecks” Technologies
Thanks to over two decades of technological development and industrialization efforts by three generations of scientists at the Chinese Academy of Sciences, the industrialization technology of PLA materials has broken foreign technology monopolies, overcoming "bottleneck" issues and achieving international leadership in the second-generation industrialization technology.
The Changchun Institute of Applied Chemistry of the Chinese Academy of Sciences, as the only research institute in China that has undergone large-scale industrial verification for PLA synthesis technology, has more than 20 years of research, development, and industrialization experience. In recent years, the Institute has conducted in-depth research and development and pilot testing to address a series of key technological and equipment issues in the PLA industry, such as the high-temperature racemization and carbonization of lactide during production, the high energy consumption and low purity in lactide purification, and the difficulties in mass and heat transfer due to the high viscosity of the polymerization reaction system. As a result, the institute has taken a leading position globally in PLA material industrialization process technology.
Key Features of PLA
The characteristic of polylactic acid is its excellent biodegradability. The biodegradability of PLA allows it to decompose into lactic acid in nature, which then hydrolyzes into carbon dioxide and water. Through photosynthesis, these elements are converted back into starch, forming a natural cycle that promotes ecological balance. Replacing conventional plastics with PLA can address the global issue of white pollution that plagues the world today. It is one of the key paths for global plastic pollution control. Vigorously developing polylactic acid materials is an important guarantee for the successful implementation of the country's "plastic restriction order".
②Biocompatibility: PLA's excellent biocompatibility has led to its widespread application in the biomedical field.
PLA
PLA is a polyester material widely recognized as a promising new material among biobased materials.
PLA is a polyester material and a recognized promising new material among biobased materials. Its raw materials come from natural biomass and have fully biodegradable properties. It can be utilized by microorganisms in nature, ultimately producing carbon dioxide and water, with no environmental pollution. PLA exhibits excellent mechanical and processing properties similar to those of existing plastics such as PET and polystyrene. Additionally, PLA has good biocompatibility, being non-toxic and non-irritating. It can be biodegraded and absorbed, and broken down by enzymes or degraded into small molecules and excreted from the body.
Bio-based
Bio-based plastics are distinguished from petroleum-based plastics by the initial source of the material.
PLA, the raw material of which comes from plants such as corn, cassava, and sugarcane, boasts an inexhaustible supply. Upon decomposition, PLA is absorbed by plants through photosynthesis, forming a green carbon cycle that can liberate us from reliance on traditional fossil materials and achieve sustainable social development. Research indicates that the lifecycle carbon emissions of PLA are more than 50% lower than those of traditional petrochemical materials. An LCA study reveals that the energy consumption during the entire production chain of every kilogram of PLA products is 64.13MJ, significantly lower than that required for producing the same amount of petroleum-based materials. Furthermore, the CO2 emissions per kilogram of PLA products are only 1.62 kilograms, far less than those of PP, PS, PET, and other petroleum-based materials. Therefore, biobased PLA materials are a key pathway to realizing the national "dual-carbon" strategy.